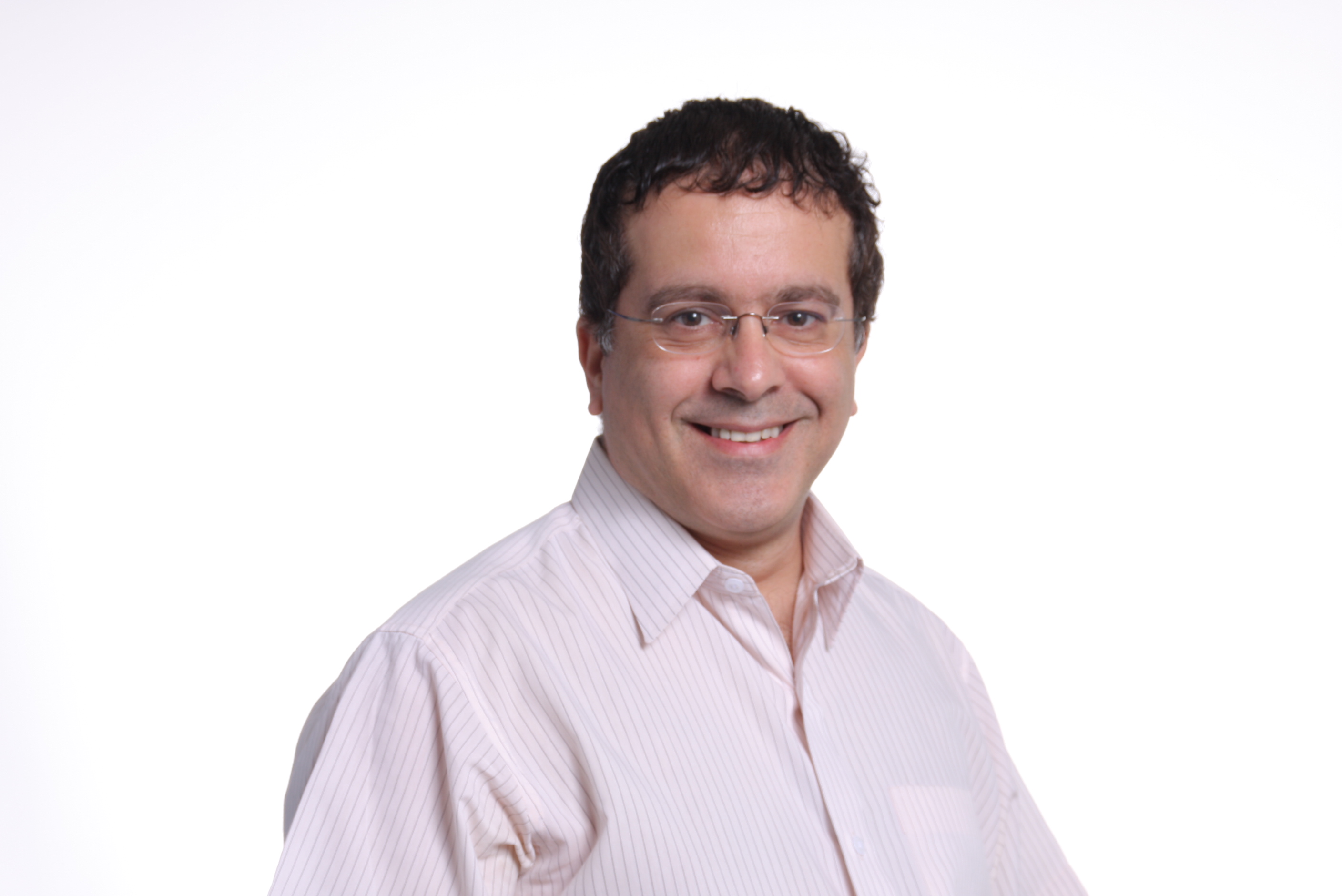
Ph.D.: Technion, Israel
Post-doctoral fellowship: Harvard University School of Medicine
Position: Associate Professor
Department of Clinical Biochemistry and Pharmacology
Faculty of Health Sciences
E-mail: Shirihai@me.com
Mitochondrial dynamics in metabolic diseases
Mitochondria have a very active social life style involving frequent fusion and fission events. Mitochondria that lose their ability to properly respire become excluded from the networking population and will be consumed by the cellular equivalent of a lion, the autophagosome. This forms a pathway of quality control. However, recent studies suggest that arrest of mitochondrial fusion at the cellular level, also termed "fragmentation", is playing a role in the adaptation to excess nutrient environment. Recognizing that excess nutrient environment places mitochondria in a biological conflict of interest may help understanding the link between metabolic and aging associated conditions.
We study two disease models in which oxidative damage to mitochondria play a key role in the development of pathology. In diabetes, nutrient-induced oxidative damage has been shown to be a major mediator of endocrine dysfunction and beta cell loss. In bone marrow, oxidative damage induced by iron and heme-intermediates, leads to the development of sideroblastic anemia and myelodysplastic syndrome.
Mitochondrial Dynamics in the beta cell: Mitochondria in β-cells play a key role as integrators of nutrient signals and insulin secretion. One significant manifestation of diabetes is the gradual reduction in mitochondrial capacity to produce signals in response to fuels. The cause of this gradual deterioration is not yet understood. Our goal is to understand the mechanisms that underlie deterioration of mitochondrial function during the development of β-cell dysfunction and diabetes.
We have shown that β-cells respond to the chronic exposure to high levels of glucose and fatty acids with a drastic reduction in mitochondrial networking through fusion and fission. This phenomenon precedes a gradual deterioration of mitochondrial function that is characterized by the generation of a subpopulation of mitochondria with reduced membrane potential. Remarkably, under these conditions, induction of mitochondrial fusion in the β-cell prevents the appearance of mitochondria with reduced membrane potential and protects from the detrimental effects of chronic exposure to a nutrient rich environment (see publications list: Molina et al. 2009).
Mitochondrial quality control: By tagging and tracking individual mitochondria in intact β-cells we discovered the existence of a quality control mechanism that relies on both fusion and fission. Following mitochondrial fission some daughter units depolarize. These units display a lower likelihood for subsequent fusion and are apparent targets of autophagy (see Twig et al. 2008). Moreover, this model predicts that the inhibition of mitochondrial dynamics (MtDy) by Gluco-lipo-toxicity (GLT) may have a cumulative effect and result in an increased portion of dysfunctional units over time. Such enrichment of dysfunctional mitochondria could explain the long lasting effect of GLT, a phenomenon that has been shown to impact animals’ prognosis many months after a high fat diet has been discontinued (see Liesa and Shirihai, 2013).
Mitochondrial Dynamics in the regulation of Energy efficiency: Brown fat is a unique tissue that can reduce its energy efficiency in response to hormonal stimulation. Upon stimulation with adrenergic agonists brown adipocyte mitochondria switch from efficient ATP producing metabolism to heat producing metabolism mediated by uncoupling. We are using this model to study the role of mitochondrial architecture and dynamics in energy efficiency. Our findings indicate that fragmented mitochondrial network enhances energy expenditure and as such may be a mechanism by which we can increase caloric consumption by the brown adipose tissue.
Twig G., Elorza A., Molina A.J., Mohamed H., Wikstrom J.D., Walzer G., Stiles L., Haigh S.E., Katz S., Las G., Alroy J., Wu M., Py B.F., Yuan J., Deeney J.T., Corkey B.E. and Shirihai O.S. (2008). Fission and selective fusion govern mitochondrial segregation and elimination by autophagy. EMBO J. 27:433-446.
Molina A.J., Wikstrom J.D., Stiles L., Las G., Mohamed H., Elorza A., Walzer G., Twig G., Katz S., Corkey B.E. and Shirihai O.S. (2009). Mitochondrial networking protects beta-cells from nutrient-induced apoptosis. Diabetes 58:2303-2315.
Las G., Sereda S., Wikstrom J.D., Twig G., and Shirihai O.S. (2011). Fatty acids suppress autophagic turnover in cells. J. Biol. Chem. 286:42534-42544.
Liesa M. and Shirihai O.S. (2013). Mitochondrial dynamics in the regulation of nutrient utilization and energy expenditure. Cell Metabolism 17:491-506.
Wikstrom J.D., Mahdaviani K., Liesa M., Sereda S.B., Si Y., Las G., Twig G., Petrovic N., Zingartti C., Graham A., Cinti S., Corkey B.E., Cannon B., Nedergaard J. and Shirihai O.S. (2014). Hormone-induced mitochondrial fission is utilized by brown adipocytes as an amplification pathway for energy expenditure. EMBO Journal 33(5):418-436.